Collaborative Research Project
- P Ashley, University of New England, Australia
- D Craw, University of Otago, New Zealand
In collaboration with
- K Hunter, J McQuillan, OU Chemistry Department
- M Tighe, UNE Agronomy and Soil Science
About the project
This project is documenting the processes involved in mobilizing antimony from mineral deposits into the environment. The project looks at similarities and differences between antimony and arsenic mobility, as arsenic typically occurs with antimony in these deposits (commonly with gold as well). The project also examines what happens to the antimony when it passes into the environment.
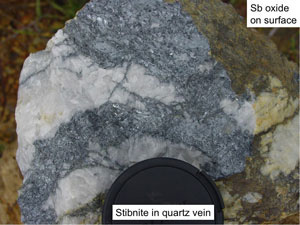
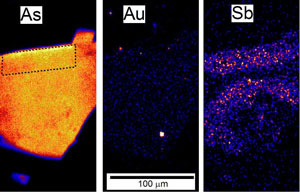
Source of antimony
The principal sources of antimony in these mineral deposits are:
- Stibnite, Sb2S3 deposited in veins with quartz (Fig. 3)
- Sb distributed through the mineral structure of arsenopyrite (FeAsS). The arsenopyrite can be in quartz veins, or in immediately adjacent rocks (Fig. 4)
Mineral deposits that have been examined as part of this programme include:
- Hillgrove antimony-gold mine, New South Wales (Figs 5,6,7)
- Endeavour Inlet historic antimony mine, Marlborough, New Zealand (Figs 8,9,10)
- Reefton gold mines, West Coast, New Zealand (Figs 11,12)
Hillgrove antimony-gold mine, New South Wales
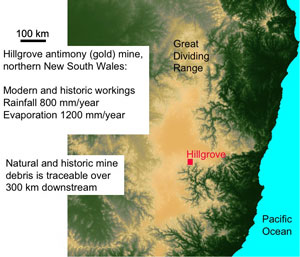
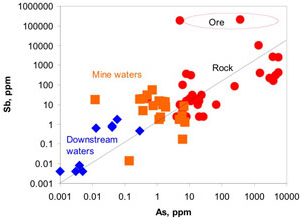
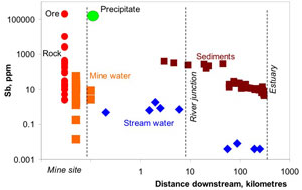
Endeavour Inlet historic antimony mine, Marlborough, New Zealand
Figure 8. Electron microprobe images (by N. Wilson) of historical (100-year old) mine tailings at Endeavour Inlet antimony mine. The images show concentrations of antimony (left) and arsenic (right). Prominent equant grains are stibnite (70 wt%) Sb) and arsenopyrite (45 wt% As). Stibnite also contains up to 5 wt% arsenic. Diffuse lower Sb and As distributions in the images are due to rainwater dissolution and redeposition associated with iron oxyhydroxide.
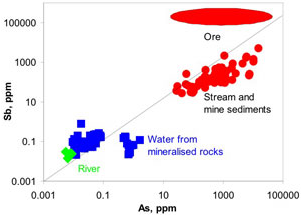
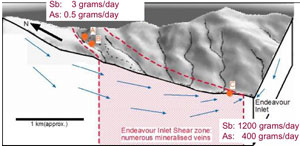
Reefton gold mines, West Coast, New Zealand
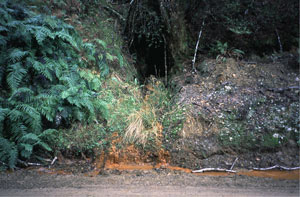
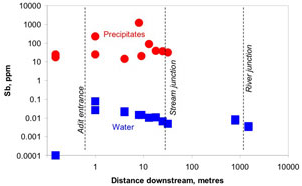
Chemical controls of antimony mobility
Sulfide minerals stibnite (Sb2S3) and arsenopyrite (FeAsS) are unstable in the presence of atmospheric oxygen. They decompose, and antimony forms oxide minerals or goes into solution.
This is summarised in the following diagram, that shows the principal chemical reactions that occur during decomposition of stibnite. The oxide minerals commonly form a white coating on the outside of stibnite samples. This white coating is soluble in rainwater and river water, up to about 50 parts per million (10,000 times drinking water limits).
Figure 13. Cartoon showing mineral transformations and chemical reactions associated with decomposition of stibnite during oxidation. Sb (iii) is antimony in the 3+ oxidation state, and Sb (v) is antimony in the 5+ oxidation state.
See also "Mobilisation and dispersal of antimony from antimony-gold mineral deposits in circumneutral pH waters: environmental consequences in Australasia" by Ashley, Craw & Tighe, presented at the International Sb Workshop in Heidelberg, Germany, May 2005
References
- Craw, D, Wilson, N & Ashley, P M. 2004. Geochemical controls on the environmental mobility of Sb and As at mesothermal antimony and gold deposits. Applied Earth Science (Trans. Inst. Min. Metall. B) V113: B3-B10.
- Wilson, N J, Craw, D. & Hunter, K. 2004. Contributions of discharges from a historic antimony mine to metalloid content of river waters, Marlborough, New Zealand. Journal of Geochemical Exploration 84: 127-139.
- Ashley, P M & Craw, D. Structural controls on hydrothermal alteration and gold-antimony mineralisation in the Hillgrove area, NSW, Australia. Mineralium Deposita 39: 223-239.
- Wilson, N J, Craw, D & Hunter, K. Antimony distribution and environmental mobility at an historic antimony smelter site, New Zealand. Environmental Pollution 129: 257-266.
- Wilson, N J, Hunter, K A, Craw, D. 2002. Elevated arsenic and antimony levels in a South Island mesothermal mineralized zone. The AusIMM 2002 Annual Conference, Auckland, New Zealand, p 81-86, Publication Series no 6/02.
Related
Introduction
Overview: Geological setting for Metals in the New Zealand environment
- Epithermal Gold
- Mesothermal Gold
- Acid Rock drainage
- Coal
- Metals in groundwater
- Alluvial Gold
- Northland Mercury
- Hot Springs (in heavy metals)
Metalloids
- Arsenic
- Antimony
- Antimony mobilisation from mineral deposits in schist and greywacke
- Mobilisation and dispersal of antimony from antimony-gold mineral deposits
- NZTEG 2008 - Antimony in the New Zealand environment